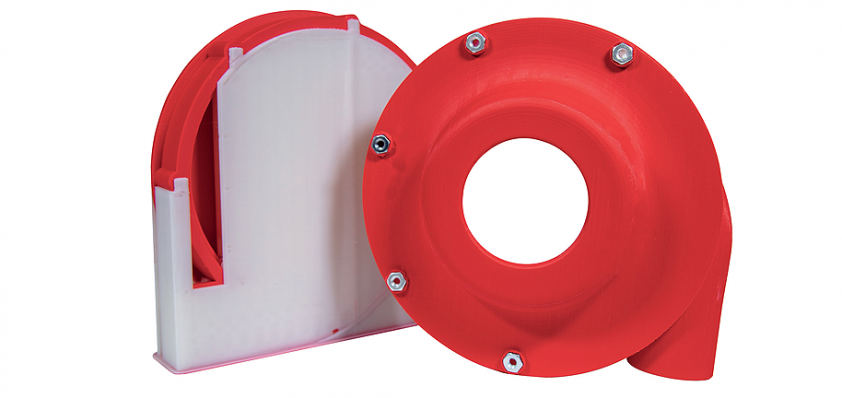
Perfecting the process
If you’ve ever had the misfortune to stand on a Lego, you’ll know how incredibly painful – not to mention indestructible – those little bricks can be. This impressive combination of durability and impressive tensile strength, not to mention the glossy finish, is due to its construction from ABS, a thermoplastic polymer widely favored for injection molded consumer goods.
In addition to its tensile strength, ABS’ impressive list of material properties include high temperature resistance, recyclability, high chemical resistance and low electric conductivity. It’s for this reason that ABS is used to make so many of the everyday items around us, from car dashboards and electrical housing, to computer keyboards and, of course, children’s toys.
While traditional production methods, such as injection molding, continue to provide the largest source of ABS parts, industrial-grade additive manufacturing has become a popular choice for the rapid production of ABS prototypes and low volume end-use parts in a cost-effective manner. While the ability to produce real engineering-grade ABS parts has become commonplace in higher-end 3D printers, desktop additive manufacturing has long struggled to produce these parts with the level of reliability and repeatability expected by an industrial designer or engineer.
The challenge
The problem stems from ABS’ high temperature resistance and melting point. When cooled this creates delamination, leading to a severe weakening of the structure of the part, and ultimately creating warping and cracks – essentially nullifying the reason for choosing ABS in the first instance. If a part is cooled rapidly by quenching, it may also be subject to shrinkage forces due to air exposure. Therefore, when producing parts in ABS using a desktop 3D printer, a controlled cooling process and closed chamber is highly recommended. The larger the part, the more likely the shrinkage forces will come into play, creating a warped or ‘taco’ shaped result.
Some engineers may abandon ABS altogether and turn to alternate materials such as PLA, which typically requires a lower print temperature of 190-230 degrees (compared to ABS’ recommended 210-250 degrees), as well as offering a reduced risk of warping. However, PLA’s low melting point comes at the price of losing large amounts of tensile strength when heated over 50 degrees, leading to a brittle part.
As engineers, we never stray from a chemical problem, and for those determined to persist with ABS, several ‘hacks’ or alternate approaches can be suggested to improve part quality. Most solutions come down to two fundamental tactics: modify the material or modify the 3D printer.
Search the internet and you’ll find an array of modified ABS materials, from thermochromic and translucent, to flame retardant and even luminous (glow in the dark). These chemical adaptations allow engineers the freedom to select the ideal formula for their specific use.
However, these altered formulas tend to come at a price, and ABS that is labelled ‘optimised for 3D printing’ is no exception. While chemically modifying ABS can reinforce heat resistance – either through the inclusion of an additive or by increasing the ratio of polybutadiene (the B in ABS) – this has to be balanced against a number of downsides: lower heat deflection temperatures, reduced tensile modulus and lower tensile strength. This can result in an inferior product that is totally unsuitable for the many of the high-performance applications ABS is used for, such as producing automotive and aerospace parts.
The evolution of accessible additive manufacturing capable of ‘real ABS’
If modifying the material doesn’t have the desired effect, then the natural progression is to look at manipulating the 3D printer itself. Many desktop 3D printers on the market today allow the user to control the temperature of the build plate. Offering a temperature-controlled heated bed allows some of the heat to be transferred to the bottom of the 3D printed part, reducing the risk of layer separation. MakerBot took this approach with its earlier 3D printers, however we found that this has limited effect. With this technique, it is not possible to consistently control the temperature of all of a part’s layers simultaneously, thereby leaving it vulnerable to warping and cracking.
When we designed the recent METHOD platform, we decided to control the temperature of the build plane, not just the build plate. Instead of simply heating from the bottom, the closed chamber allows heat to be recirculated in the chamber, pulling air across from both sides. This creates superior control as every layer is printed in the same heat environment without the need to ‘tinker’ with printer settings. This technology is designed to help engineers achieve dimensionally accurate, production-grade ABS parts at a significantly lower cost than traditional manufacturing.
Even with a new approach to controlling the build plane temperature, there are still challenges to contest with. As the extruder is in a hotter environment, there is a risk of expansion. This begs the question, if there printer, then why are we so determined to fight against these material properties?
The answer lies in our vision for the future manufacturing industry, which is that of a decentralised, on-demand production model driven by additive manufacturing. Injection molded ABS will remain the best choice for mass production for many years to come due to its speed and low-cost, but when the volume needed is in the tens, hundreds or even thousands, or customised production is required, additive manufacturing really has the upper hand. The traditional cost-benefit analysis that typically comes with creating a tool, prototype or end-use part is disrupted. Designs can be tested and iterated faster, speeding up innovation and time-to-market, all at a much lower cost than traditional methods.
The ability to use real engineering-grade ABS on more than just traditional 3D printers opens up these inherent benefits of additive manufacturing to a much wider audience of engineers, who have been limited to costly large-scale industrial 3D printers to meet their ABS requirements. This is why the recently launched METHOD X 3D printer signifies a step-change for industrial-grade additive manufacturing. Offering true ABS for the first time on a more accessible 3D printer, it enables your everyday designer or engineer to have access to engineering-grade ABS for more accurate and functional prototypes, as well as more robust and reliable production parts.
All Axis Robotics is a great example of what can be achieved with real ABS-enabled additive manufacturing. In order to adapt its custom robot end-effector designs to the shop floor, the company needed to produce a customised ABS part-sander. Using METHOD X, within a matter of hours the team was able to produce the sander using highly tough and durable ABS materials, avoiding the high costs and long lead times typically incurred using external supplier
With the importance of ABS in production never greater, it’s important that developments in additive manufacturing can extend this capability to the wider industry as an alternative to traditional methods. While more costly large-scale industrial 3D printers still remain an important tool for addressing certain industry requirements for ABS, there is no doubt that a rise in more accessible 3D printers offering real engineering-grade ABS can open up a plethora of opportunities to a much larger audience of engineers.
Dave Veisz
Dave Veisz is VP of Engineering at MakerBot. As a global leader in the 3D printing industry. MakerBot strives to redefine the standards for 3D printing for reliability, accessibility, precision, and ease-of-use. MakerBot has one of the largest install bases in the industry and also runs Thingiverse, the largest 3D printing community in the world.
www.makerbot.com